Rheology and Gelling Behavior of Boehmite Sols
M.A. Islam, Chu Chi Ming, Pogaku Ravindra and Eng-Seng Chan
Rheological studies of boehmite sols based on pH was (1) to study the nature of flow properties of the boehmite sols and (2) to identify the sol-gel transition of boehmite sols with the change of pH of the liquid. It is evident that the shear rate and viscosity of the liquid exhibit a pseudoplastic (shear thinning) flow behavior for all pH level. The transformation of sol-gel at low pH probably due to the hydrogen bond between the layers of the boehmite structure. The temperature has an appreciable effect on the activation energy during the sol-gel transition. The flow activation energies ΔEη are in the range of 1.75 to 6.25 J mol-1 at temperature 298-384 K. Based on these results, the flow activation energy of boehmite sols sharply decreases with decreasing the pH. The flocculation of the suspension at pH 4 to 1 showed to be favourable in term of enhanced density of boehmite.
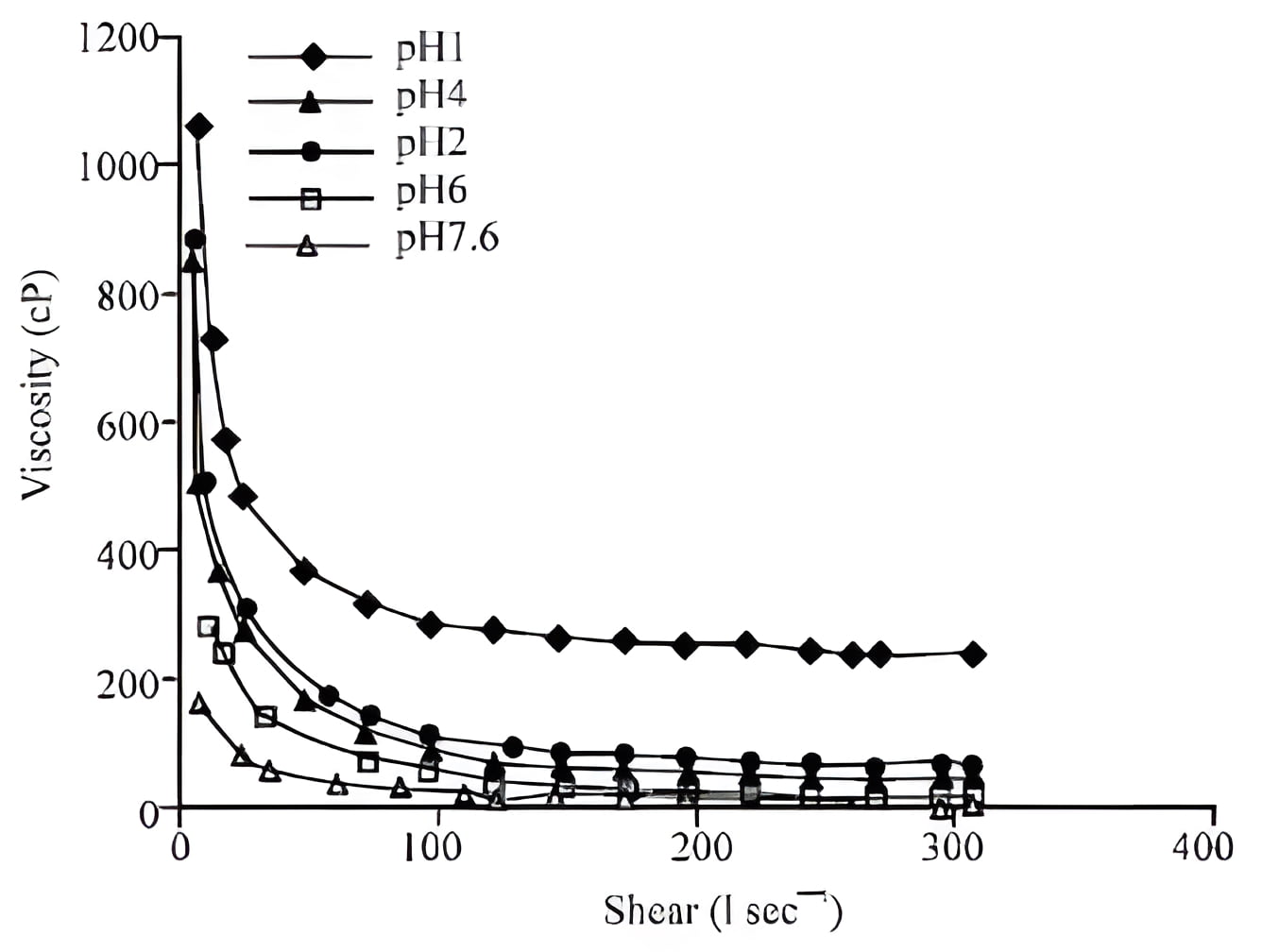
INTRODUCTION
Boehmite (AlOOH), a well known aluminium oxihydroxide has found important applications in the preparation of catalysts and catalyst support used in heterogeneous catalysis (Baumann et al., 2005; Kotanigawa et al., 1981; Hellgardt and Chadwick, 1998; Knozinger and Ratnasamy, 1978). Recently, many researchers have been focused on the synthesis of boehmite particles due to the promising foresight of their use in catalytic applications (Caiut et al., 2009; Zhang et al., 2009; Yang, et al., 2009a,b; Dash et al., 2009; Lesaint et al., 2008; Lepot et al., 2008; Guo et al., 2008). Especially, because of its large surface area, low cost, thermal stability, good mechanical strength and volatile acidity, γ-Al2O3 has been utilized as a carrier for catalyst in petroleum refining and petrochemical industries (Okada et al., 2003; Park et al., 2003; Piqueras et al., 2006a, b; Jun-Cheng et al., 2006). A number of research studies dealing with transesterification reactions catalysed by Al2O3 have been reported in the literature while many papers are devoted to esterification reaction with heterogeneous catalyst (Umdu et al., 2009; Noiroj et al., 2009; Vyas et al., 2009). The physical properties such as porosity, surface area of a catalyst hold promise for increasing the performance of catalyst in petrochemical industries (Mbaraka and Shanks, 2006; Ngamcharussrivichai et al., 2007). For this reason, the successful synthesis of mesoporous aluminas with a high surface area, a narrow pore size distribution and a high pore volume is currently searched for (Ejka, 2003).
The catalytic performance that markedly affect the characteristics of an aqueous suspension include solids loading, pH, viscosity, density , gelling nature of the liquid and concentration of the dispersant (Bleier and Westmoreland, 1991). Kim et al. (2008) evaluated the synthesize γ-Al2O3 catalysts with different surface areas by varying preparation parameters systematically during sol-gel synthesis and to correlate the catalytic activity with the surface area of γ-Al2O3. It was demonstrated that the changes in the rates of catalytic reaction could be intentionally used to obtain a particular type of gel structure and physical properties (Brinker, 1988; Klein, 1985). Additionally, the type of catalyst has a decisive role on the type of mechanism involved in the sol-gel process and the final properties of the gel (Ying et al., 1993; Zarzycki, 1990). Clearly, the type of catalyst and pH cannot be chosen indiscriminately because of their role on the final morphology, densification and physical properties. The formation of different concentrated gels of significantly different liquid properties might be achieved to increase the catalytic performance (Knozinger and Ratnasamy, 1978; Jiratova and Beranek, 1982). As a consequence, to optimize the origin of these properties it is necessary to know in details of the rheological properties of the liquid, gelling behavior and effect of pH on it which make it to establish a relation between liquid properties and catalytic performance of the boehmite sol. To identify the evolution of catalytic properties, liquid properties with well known performance of the catalyst is crucial to make out. The main restrictions for using the catalyst are associated with the lack of knowledge about many of the relation between the liquid and the physical properties which influence the catalytic performance. To bridge this gap, we study the liquid properties of boehmite systemically and the results are being presented in this study what, to our knowledge has not been yet studied.
MATERIALS AND METHODS
Boehmite (AlOOH) powders were produced by BASF chemical company (G-250, medium density pseudoboehmite alumina, BASF Catalysts LLC in USA, purity>99%). The sodium hydroxide were of analytical grade. Different amounts of Boehmite (AlOOH, BASF) ranging from 3 to 50 g, were dispersed separately in 100 mL of deionized water, the suspension being dispersed under ultrasounds for 2 min and then stirred for 20 min. The sols were further acidified as pH (1.0-7.6) at 25°C by the addition of concentrated hydrochloric acid. Table 1 shows the chemical composition of AlOOH.
The particle size distribution of alumina powder was measured by Particle Analyzer (HORIBA LA-300-Laser Scattering Particle Size Distribution Analyzer, Japan). The viscosity of the boehmite was measured using viscometer model LV-DV III+ (Brookfield Engineering Laboratories, Inc., USA) at different of spindle speeds in rpm. The readings were taken only after 5 min of revolution. The appropriate disc spindle was selected so that the torque readings were not below 10% of the total scale. The apparatus is designed to measure rheological parameters of shear stress and viscosity at given shear rates. The rheometer was connected to a circulating water bath (Brookfield Refrigerated Bath/Circulator Model TC-500, Brookfield Engineering Laboratories, Inc., Stoughton, MA 02072-2398 USA) for precise temperature control. The liquid density was measured at 25°C by using a digital density/specific gravity meter (Kyoto Electronics Manufacturing Co Ltd, Japan). The pH of the liquid was measured by pH meter at 25°C using pH meter (Cyber Scan pH 1500, Eutech Instruments Pte Ltd, Singapore).
Table 1: The chemical compositions of AlOOH
Sonication of the suspension was performed by high intensity ultrasonic processor with temperature controller microprocessor controlled (750 Watt model).
RESULTS AND DISCUSSION
Flow behavior: Measurements were performed within the range of shearing rate from 10 to 306 sec-1, while the dynamic viscosity ranged from 4 to 1200 cP at a temperature of 25°C. Experimental data showing variation of viscosity with shear rate for the boehmite slurries at different pH are illustrated in Fig. 1 The dynamic apparent viscosity decreases exponentially on increasing the shear rate which is remarkable for all the investigated solution pH. It is evident that the liquid exhibit a pseudoplastic (shear thinning) flow behavior for all pH level. As a matter of fact, strong shear dependence is observed. At the same shear rate, the apparent viscosities of low pH is higher than the viscosities of higher pH. The viscosity decreases less than proportionally with the increase of shear rate. The flow properties of boehmite sols under acidic and basic conditions shows shear thinning/non-Newtonian pseudoplastic behaviour that might be the functions of particle-particle aggregation due to weak forces of attraction (e.g. VanderWaals forces) Fig. 2. It can be explained by reason that the aggregates of particles are formed just after lowering the pH and broken into pieces at high shear rates results in shear thinning behavior.
Flow activation energy: For further understanding the sensitivity of viscosity to temperature at different pH, the flow activation energy (ΔEη) is deduced by Arrhenius equation (Eq. 1):
(1)
A is the temperature independent pre-exponential term or the frequency factor, R the gas constant (8.314 J/mol K ) and T the reaction temperature in Kelvin. Taking the logarithm of Eq. 2 and plot of log (η) vs. 1/T should be linear with a slope of (ΔEη/R). The solid lines in the Fig. 3 obtained from linear least-squares fitting of the experimental data to Eq. 1.
Table 2 gives the values of activation energy at zero shear rate for different pH at various temperatures. The Arrhenius equation indicates the general tendency observed for an apparent viscosity decrease with a temperature increase. In general the greater the activation energy the greater the temperature effect on viscosity. This is evident from the viscosity values observed for boehmite sols, which vary significantly from 25 to 75°C.
Fig. 1: The particle size distribution of Boehmite powder
Fig. 2: Viscosity versus shear rate plot for 30 % (w/v) boehmite for different pH at 25°C
Fig. 3: Viscosity at zero shear rate of boehmite solution sols of 30% (w/v) at different pH as a function of temperature
The results revealed that acid catalysed system (pH 1) had a ΔE of 1.75 J mol-1 and base catalysed system (pH 7.6) h ad a ΔE of 6.25 J mol-1. The main purpose of a catalyst in a chemical reaction is to lower the activation energy of a reaction so that it can occur at a lower temperature or to accelerate the reaction (Laidler, 1987; Chang, 1988; Huheey et al., 1993).
Table 2: Influence of pH on gelation at various temperatures (30% (w/v) of boehmite sol
The flow activation energy of boehmite sols sharply decreases with decreasing pH. This seems to be attributed to the participation of the H+ ions in the liquid to form a gel network (M-O-M) during the condensation process that may decrease the value of E.
Brinker et al. (1982) demonstrated that the temperature can control the gelation process by modifying the rates of hydrolysis and condensation. Livage et al. (1988) proposed hydrolysis and condensation mechanism through transition hydrogen bonds according to Eq. 2 and 3. The relative different in bond strength between the ionisable species and molecule may determine the activation energy condensation process. The reactions produce large molecular weight molecules that continuously combine to form an infinite molecular weight network that characterized by gel-point (Sperling, 1992; Munk, 1989; Odian, 1981). The gel point occurs when an infinite molecular weight molecule is formed throughout the reaction medium. The H+ ions may bond weakly to the boehmite gel network:
or may have a tendency to mobile in the gel with respect to temperature at low pH. As the H+ ions become part of the gel network, they are not mobile anymore and have tendency to form gel at low temperature. The morphology formed during the condensation of the sols has a direct relationship to the final properties of the gel (Ying et al., 1993; Zarzycki, 1990; Brinker et al., 1982; Schmidt et al., 1984):
(2)
(3)
Sol-Gel transformation: Sol-gel is the process in which a sol agglomerates to form a gel. In this study, the influences of pH on the zero-shear viscosity of boehmite dissolved in water is depicted in Fig. 4. The curve shows similar trends in their viscosity values with respect to pH of boehmite sols. The viscosity remains almost the same as the pH decreases from 7.6 to 4 and then increases sharply which might be indicative a tendency of gelation. The boehmite sols shows the lowest viscosity values at the pH of 4 to 1 indicative of these as the regions of best dispersion of boehmite particles for all concentration which lowers the viscosity a couple of orders of magnitude (Fig. 4). The decrease of viscosity is due both to the decrease of gel strength and to the re-dispersion of the flocks. Small changes of viscosity in dispersion region might be indicating no intermolecular interactions owing to electrostatic repellence.
With the progressive decrease of pH value from 4.0 to 1.0, the liquid exhibit a marked viscosity enhancement suggesting that the electrostatic repellence are suppressed and enhanced the intermolecular bonds. It is probably an indication of the high levels of agglomeration of particles. Boehmite is not retain well disperse at pH 4 results in partly flocculated and it becomes fully flocculated at pH 1 where the attractive interparticle potential dominates. The gelling behavior of boehmite may rise the cohesiveness of the liquid to increase the viscosity. It is reported that higher viscosity observed at very highly acidic (pH1±2) conditions is the characteristic of flocculated suspensions (Yang et al., 2009a). A rapid development of particle-particle interconnectivities may take place during lowering the pH. The viscosity relates to the pH when particles tends to pack together, making flow impossible (Cristiani et al., 2005; Leenaars et al., 1984; Yoldas, 1975). The viscosity increases up to the maximum level due to the growing amounts of gel, to the increase of the gel strength and to the formation of the flocks that make it clear to indentified the transition of sol to gel of the liquid. Additionally, Gel formation is associated with rapid increase in viscosity and observations of a maximum viscosity (Sakka and Kamiya, 1982; Nogami et al., 1980). The adhesion of aggregates extends out through the whole suspension and can give solid like gel structures.
Fig. 4: Effect of pH on viscosity at zero shear rate for different concentration at 25°C
However, continuous decreasing pH brings particles closer, stronger interaction and finally a sufficiently developed gel structure. Brinker and Scherer (1990) reported that catalyst type and pH of the reaction determines the resulting morphology and growth model in sol-gel chemistry. Fast hydrolysis and slow condensation rates characterize an acidic liquid which produces clustered structures that is described by a cluster-cluster growth models (Brinker and Scherer, 1990; Schaefer, 1988). These limitations in the condensation reaction result in a variety of structure for acidic liquid system. Because the reaction conditions, the final mechanical properties are dependent on whether the skeleton structure is produced from linear or cluster structure (Brinker and Scherer, 1990). The final microstructure that evolves acidic or basic liquid is schematically represented in Fig. 5.
From Fig. 4 and 5, it is apparent that the liquid properties are extremely important for the production of catalyst. In order to gain additional insight on the affect of pH, Ying et al. (1993) found that acid catalyzed gels had significantly higher porosity and dimensional shrinkage. It can be demonstrated that catalyst and pH actually control the final gel properties by influencing microstructure of the gel. The degree of structure formation such as agglomeration, flocculation and the strength of the interparticle binding forces is manifested by the limit of gel formation of the liquid. Munk (1989) reported that the viscosity increase has been associated with the increase of the attraction forces among the colloidal agglomerates or progressive aggregation of particles that implies gelation and a branched cluster structure. Therefore, the gelling behavior affect the shape, size and porous characteristics of boehmite sols that are important factor for better performance of catalyst.
Fig. 5: Schematic representation of an arbitrary gel densification from or base or acid catalysed system (a) Linear or randomly branched gel structure and (b) highly clustered structure
Fig. 6: Density of boehmite as a function of pH
Effect of density on pH: The effect of pH on density of different wt.% (w/v) boehmite sols exhibits considerable differences as seen in Fig. 6. Based on experiments, it is demonstrated that pH of the boehmite is responsible for changing the density of the liquid. Surprisingly, high density was found at low pH even in the low concentration. Kitayama and Pask (1996) reported the alumina powder reacts in an acid solution to form a gel and consequently formed hard agglomerates. Boehmite may form agglomerate by condensation of dissolved aluminum species at interparticle contacts during the formation of gel. If gel is formed by precipitation of the dissolved species from solution, the bonding between particles will be much coherent and stronger that might responsible for increase the density of solution.
The influence of pH may affect the interparticle bonding forces of the boehmite sols and subsequently increase the density depending on the nature of the interparticle force (Kwon and Messing, 1997). Of these bonding mechanisms, we believe that chemical bonding is the reason for the higher density of the water-dispersed boehmite agglomerates. The solubility of the material itself may be the reason for agglomerate density (Brinker and Scherer, 1990). Therefore, either electrostatic forces or Vander Waals forces are considered to be responsible for increasing the density of the gel (Kwon and Messing, 1997). Liquid densities for acidic and basic liquid will vary depending on the initial reaction condition but it can be generalized that basic liquid are always lower in density than their acidic liquid. Since gelling on the alumina surface depends on the population and the ionization status of hydroxyl groups presented on the alumina surface, this suggests the electrostatic interaction between boehmite particles to play a dominant role in determining the density behavior of boehmite even when the pH of system is high (Chen et al., 1998). This is attributed to pH dependence of possible hydrogen bonding as a result of the pH dependent ionization of hydroxyl groups on boehmite. This can be accounted by the enhancement of particles interaction that are closer to each other at low pH. Correlation between the suspension rheology and the density of boehmite sol suggests that increased interaction between particles at low pH to be a possible reason higher density.
CONCLUSION
The rheological properties of boehmite sols under acidic and basic conditions shows shear thinning/non-Newtonian pseudoplastic behaviour that might be the functions of particle-particle aggregation due to weak forces of attraction (e.g., Vander Waals forces). A rapid development of particle-particle interconnectivities may take place during lowering the pH. Gelation activation energy (ΔEη) is dependent of the boehmite concentration and pH of the solution. Based on the results, the temperature can control the gelation process by modifying the rates of hydrolysis and condensation to increase value of E. The sol-gel transition of boehmite sol is identified by the pH shows that the viscosity increases up to the maximum level due to the growing amounts of gel, to the increase of the gel strength and to the formation of the flocks. Correlation between the suspension rheology and the density of boehmite sol suggests that increased interaction between particles at low pH to be a possible reason for higher density.
ACKNOWLEDGMENT
The authors wish to thank Universiti Malaysia Sabah (UMS) for funding the project under research grant code SCF0034-IND-1/2007.
REFERENCES
1: Baumann, T.F., A.E. Gash, S.C. Chinn, A.M. Sawvel, R.S. Maxwell and J.H. Satcher Jr., 2005. Synthesis of high-surface-area alumina aerogels without the use of alkoxide precursors. Chem. Mater., 17: 395-401.
CrossRef |
2: Kotanigawa, T., M. Yamamoto, M. Utiyama, H. Hattori and K. Tanabe, 1981. The influence of preparation methods on the pore structure of alumina. Applied Catal., 1: 185-200.
CrossRef |
3: Hellgardt, K. and D. Chadwick, 1998. Effect of pH of precipitation on the preparation of high surface area aluminas from nitrate solutions. Ind. Eng. Chem. Res., 37: 405-411.
CrossRef |
4: Knozinger, H. and P. Ratnasamy, 1978. Catalytic aluminas: Surface models and characterization of surface sites. Catal. Rev., 17: 31-70.
CrossRef |
5: Caiut, J.M.A., J. Dexpert-Ghys, Y. Kihn, M. Verelst, H. Dexpert, S.J.L. Ribeiro and Y. Messaddeq, 2009. Elaboration of boehmite nano-powders by spray-pyrolysis. Powder Technol., 190: 95-98.
CrossRef |
6: Zhang, L., W. Lu, L. Yan, Y. Feng and X. Bao et al., 2009. Hydrothermal synthesis and characterization of core/shell ALOOH microspheres. Microporous Mesoporous Mater., 119: 208-216.
CrossRef |
7: Yang, J., R.L. Frost and Y. Yuan, 2009. Synthesis and characterization of chromium doped boehmite nanofibres. Thermochim. Acta, 483: 29-35.
CrossRef |
8: Dash, B., B.C. Tripathy, I.N. Bhattacharya, S.C. Das, C.R. Mishra and B.K. Mishra, 2009. Precipitation of boehmite in sodium aluminate liquor. Hydrometallurgy, 95: 297-301.
CrossRef |
9: Lesaint, C., W.R. Glomm, O. Borg, S. Eri, E. Rytter and G. Oye, 2008. Synthesis and characterization of mesoporous alumina with large pore size and their performance in Fischer-Tropsch synthesis. Applied Catal. A: Gen., 35: 131-135.
CrossRef |
10: Lepot, N., M. van Bael, H. van den Rul, J. D’Haen, R. Peeters, D. Franco and J. Mullens, 2008. Synthesis of platelet shaped boehmite and gamma-alumina nanoparticles via an aqueous route. Ceram. Int., 34: 1971-1974.
11: Guo, X.K., P.P. Xie and S.D. Lin, 2008. Preparation of Cu/La2O3-ZrO2-Al2O3 catalyst and its catalytic properties for selective reduction of NO. J. Fuel Chem. Technol., 36: 732-737.
CrossRef |
12: Okada, K., T. Nagashima, Y. Kameshima and A. Yasumori, 2003. Effect of crystallite size of boehmite on sinterability of alumina ceramics. Ceram. Int., 29: 533-537.
CrossRef |
13: Park, T.J., J.S. Lim, Y.W. Lee and S.H. Kim, 2003. Catalytic supercritical water oxidation of wastewater from terephthalic acid manufacturing process. J. Supercrit. Fluids, 26: 201-213.
CrossRef |
14: Piqueras, C., S. Bottini and D. Damiani, 2006. Sunflower oil hydrogenation on Pd/Al2O3 catalysts in single-phase conditions using supercritical propane. Applied Catal. A: Gen., 313: 177-188.
CrossRef |
15: Piqueras, C.M., M.B. Fernandez, G.M. Tonetto, S. Bottni and D.E. Damiani, 2006. Hydrogenation of sunflower oil on Pd catalysts in supercritical conditions: Effect of the particle size. Catal. Commun., 7: 344-347.
CrossRef |
16: Jun-Cheng, L., X. Lan, X. Feng, W. Zhan-Wen and W. Fei, 2006. Effect of hydrothermal treatment on the acidity distribution of γAl2O3 support. Applied Surf. Sci., 253: 766-770.
CrossRef |
17: Umdu, E.S., M. Tuncer and E. Seker, 2009. Transesterification of Nannochloropsis oculata microalga’s lipid to biodiesel on Al2O3 supported CaO and MgO catalysts. Bioresour. Technol., 100: 2828-2831.
CrossRef | Direct Link |
18: Noiroj, K., P. Intarapong, A. Luengnaruemitchai and S. Jai-In, 2009. A comparative study of KOH/Al2O3 and KOH/NaY catalysts for biodiesel production via transesterification from palm oil. Renewable Energy, 34: 1145-1150.
CrossRef | Direct Link |
19: Vyas, A.P., N. Subrahmanyam and P.A. Patel, 2009. Production of biodiesel through transesterification of Jatropha oil using KNO3/Al2O3 solid catalyst. Fuel, 88: 625-628.
CrossRef |
20: Mbaraka, I.K. and B.H. Shanks, 2006. Conversion of oils and fats using advanced mesoporous heterogeneous catalysts. J. Am. Oil Chem. Soc., 83: 79-91.
CrossRef | Direct Link |
21: Ngamcharussrivichai, C., W. Wiwatnimit and S. Wangnoi, 2007. Modified dolomites as catalysts for palm kernel oil transesterification. J. Mol. Catal. A: Chem., 276: 24-33.
CrossRef |
22: Ejka, J.C., 2003. Organized mesoporous alumina: Synthesis, structure and potential in catalysis. Applied Catal. A: Gen., 254: 327-338.
CrossRef |
23: Bleier, A. and C.G. Westmoreland, 1991. Effects of pH and particle size on the processing of and the development of microstructure in alumina-zirconia composites. J. Am. Ceram. Soc., 74: 3100-3111.
CrossRef |
24: Kim, S.M., Y.J. Lee, J.W. Bae, H.S. Potdar and K.W. Jun, 2008. Synthesis and characterization of a highly active alumina catalyst for methanol dehydration to dimethyl ether. Applied Catal. A: Gen., 348: 113-120.
CrossRef |
25: Brinker, C.J., 1988. Hydrolysis and condensation of silicates: Effects on structure. J. Non-Cryst. Solids, 100: 31-50.
CrossRef | Direct Link |
26: Klein, L.C., 1985. Sol-gel processing of silicates. Annu. Rev. Mater. Sci., 15: 227-248.
CrossRef |
27: Ying, J.Y., J.B. Benziger and A. Navrotsky, 1993. Structural evolution of alkoxide silica gels to glass: Effect of catalyst pH. J. Am. Ceram. Soc., 76: 2571-2582.
CrossRef |
28: Zarzycki, J., 1990. Structural aspects of sol-gel synthesis. J. Non-Cryst. Solids, 121: 110-118.
CrossRef |
29: Jiratova, K. and L. Beranek, 1982. Properties of modified aluminas. Applied Catal., 2: 125-138.
CrossRef |
30: Laidler, K.J., 1987. Chemical Kinetics. 3rd Edn., Harper Collins Publishers, New York, ISBN-13: 9788131709726, pp: 544
31: Chang, R., 1988. Chemistry. 3rd Edn., Random House Inc., New York, USA
32: Huheey, J.E., E.A. Keiter and R.L. Keiter, 1993. Inorganic Chemistry Principles of Structure and Reactivity. 4th Edn., Prentice Hall, USA., ISBN-13: 978-0060429959
33: Brinker, C.J., K.D. Keefer, D.W. Schaefer and C.S. Ashley, 1982. Sol-gel transition in simple silicates. J. Non-Cryst. Solids, 48: 47-64.
CrossRef |
34: Livage, J., M. Henry and C. Sanchez, 1988. Sol-gel chemistry of transition metal oxides. Prog. Solid State Chem., 18: 259-341.
CrossRef |
35: Sperling, L.H., 1992. Introduction to Physical Polymer Science. John Wiley and Sons, New York
36: Munk, P., 1989. Introduction to Macromolicular Science. John Wiley and Sons, New York, USA
37: Odian, G., 1981. Principles of Polymerization. 2nd Edn., John Wiley and Sons, New York
38: Yoldas, B.E., 1975. Alumina gels that form porous transparent Al2O3. J. Mater. Sci., 10: 1856-1860.
CrossRef |
39: Schmidt, H., H. Scholze and A. Kaiser, 1984. Principles of hydrolysis and condensation reaction of alkoxysilanes. J. Non-Cryst. Solids, 63: 1-11.
CrossRef |
40: Yang, J., S. Chen and Y. Fang, 2009. Viscosity study of interactions between sodium alginate and CTAB in dilute solutions at different pH values. Carbohydr. Polym., 75: 333-337.
CrossRef |
41: Cristiani, C., M. Valentini, M. Merazzi, S. Neglia, P. Forzatti, 2005. Effect of ageing time on chemical and rheological evolution in γ-Al2O3 slurries for dip-coating. Catal. Today, 105: 492-498.
CrossRef |
42: Leenaars, A.F.M., K. Keizer and A.J. Burggraaf, 1984. The preparation and characterization of alumina membranes with ultra-fine pores. Part 1 Microstructural investigations on non-supported membranes. J. Mater. Sci. Eng., 19: 1077-1088.
CrossRef |
43: Sakka, S. and K. Kamiya, 1982. The sol-gel transition in the hydrolysis of metal alkoxides in relation to the formation of glass fibers and films. J. Non-Cryst. Solids, 48: 31-46.
CrossRef |
44: Nogami, M. and Y. Moriyaa, 1980. Glass formation through hydrolysis of Si(OC2H5)4 with NH4OH and HCl solution. J. Non-Cryst. Solids, 37: 191-201.
CrossRef |
45: Brinker, C.J. and G.W. Scherer, 1990. Sol-Gel Science: The Physics and Chemistry of Sol-Gel Processing. 1st Edn., Academic Press, San Diego, ISBN-13: 978-0121349707, pp: 912
46: Schaefer, D.W., 1988. Fractal models and the structure of materials. MRS Bull., 8: 22-27.
47: Kitayama, M. and J.A. Pask, 1996. Formation and control of agglomerates in alumina powder. J. Am. Ceram. Soci., 79: 2003-2011.
CrossRef |
48: Kwon, S. and G.L. Messing, 1997. The effect of particle solubility on the strength of nanocrystalline agglomerates: Boehmite. Nanostruct. Mater., 8: 399-418.
CrossRef |
49: Chen, T.Y., C. Maltesh and P. Somasundaran, 1998. Effect of Solids Concentration on Polymer Adsorption and Conformation. Plenum Press, New York